FRET-based tension sensor
All organisms in nature are mechanosensitive. As the basic unit of structure and function of prokaryotes and eukaryotes, cells’ inherent capabilities to sense external forces and transmit them are important to understand in the context of basic biophysics, but also eventually for human health and disease. Many molecular mechanisms have been identified to play crucial roles in driving and regulating cell activities. However, further progress has been hampered by the fundamental difficulty to measure stresses in cells. Being able to determine which proteins and lipids bear load is critical to understand mechanosensitive signaling and regulatory control of local force generation. The cytoskeleton, a dynamic protein polymer network, is the most essential load-bearing and force-producing subcellular structure. In this project, we focus on forces transmitted through of one its major components—the actin network and will use actin-targeting tension sensors, developed by Prof. Brent Hoffman’s lab at Duke University, to study and understand the way forces are propagating within this subcellular structure and the mechanical loads experienced by crosslinking proteins within the actin cortex. The novel aspect of my thesis work is that I will directly apply controlled forces to cells to observe force propagation and that I will use concepts of polymer physics to interpret the data, looking for time-dependent percolation effects, formation of force chains in the semiflexible, and nonlinear polymer networks.

Mechanical properties of suspended cell
The mechanical properties of most animal cells are dominated by the actin cortex, a~ 0.5 µm thick layer of actin filaments including a multitude of associated proteins, which is attached to the inner side of the cell membrane and encapsulates the cytoplasm. Cells can actively change their shape and volume, but osmotic pressure prohibits any substantial volume change in response to external forces.

right ) 3T3 fibroblast cell suspended between two optically trapped 4 µm fibronectin-coated polystyrene beads.
We have mechanically indented suspended spherical with dual optical tweezers set-up to measure response. For small, slow indentations we find a linear elastic response. We use finite element simulation for modeling the cell as an elastic shell.
Flow and mechanosensing via primary cilia in kidney epithelial cells
Many cell types are mechanosensitive, which is important for communication between cells and their environment. Mechanosensitivity involves membrane channels and coupled cytoskeletal structures. Defects in mechanosensing have been linked to human diseases, such as polycystic kidney disease (PKD). We study: (i) model systems
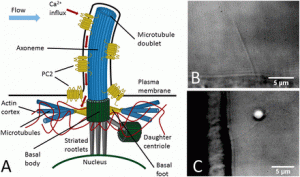
of substrate-supported lipid membranes with embedded channels and attached polymer networks to study basic mechanical properties and channel activation, (ii) cells that sense flow, with a focus on the role of primary cilia and TRP channels. We will use optical trapping, microrheology, electrical recording, and fluorescence microscopy.
Mechanosensing in bone cells

Specialized cells inside the bone matrix, the osteocytes, are the detectors of mechanical stress and strain and chemically signal to other cells in a complex regulatory network controlling the dynamic remodeling of bone. Understanding this regulatory system is medically important to fight age-related osteoporosis, but also for bone healing after injury, for implant surgery, dental surgery, and even to solve health problems humans encounter during space missions. We are developing methods to directly mechanically stimulate single osteocytes, to study their mechanical properties and measure their chemical signalling response, in particular the release of nitric oxide. (collaboration with J. Klein-Nulend, T. Smit, VUMC Amsterdam, Oxford)
Protective mechanisms and mechanosensing of vascular endothelial cells

The cells lining the inside of blood vessels need to protect themselves against damage by blood flow itself and the potentially dangerous molecular contents of blood (e.g. cholesterol). It is generally believed that a highly dynamic polyelectrolyte layer on the surface of the cells, the glycocalix, is responsible for this protection and its breakdown appears to stand at the beginning of many vascular and heart diseases. Regulation of the glycocalix occurs through mechanosensing again, possibly by similar mechanisms as in the bone cells. We are studying the viscoelastic properties of the glycocalix in cultured cells and we are exploring possibilities to study the dynamic properties of this extracellular matrix inside of blood vessels. (collaboration with J. Spaan, AMC Amsterdam)